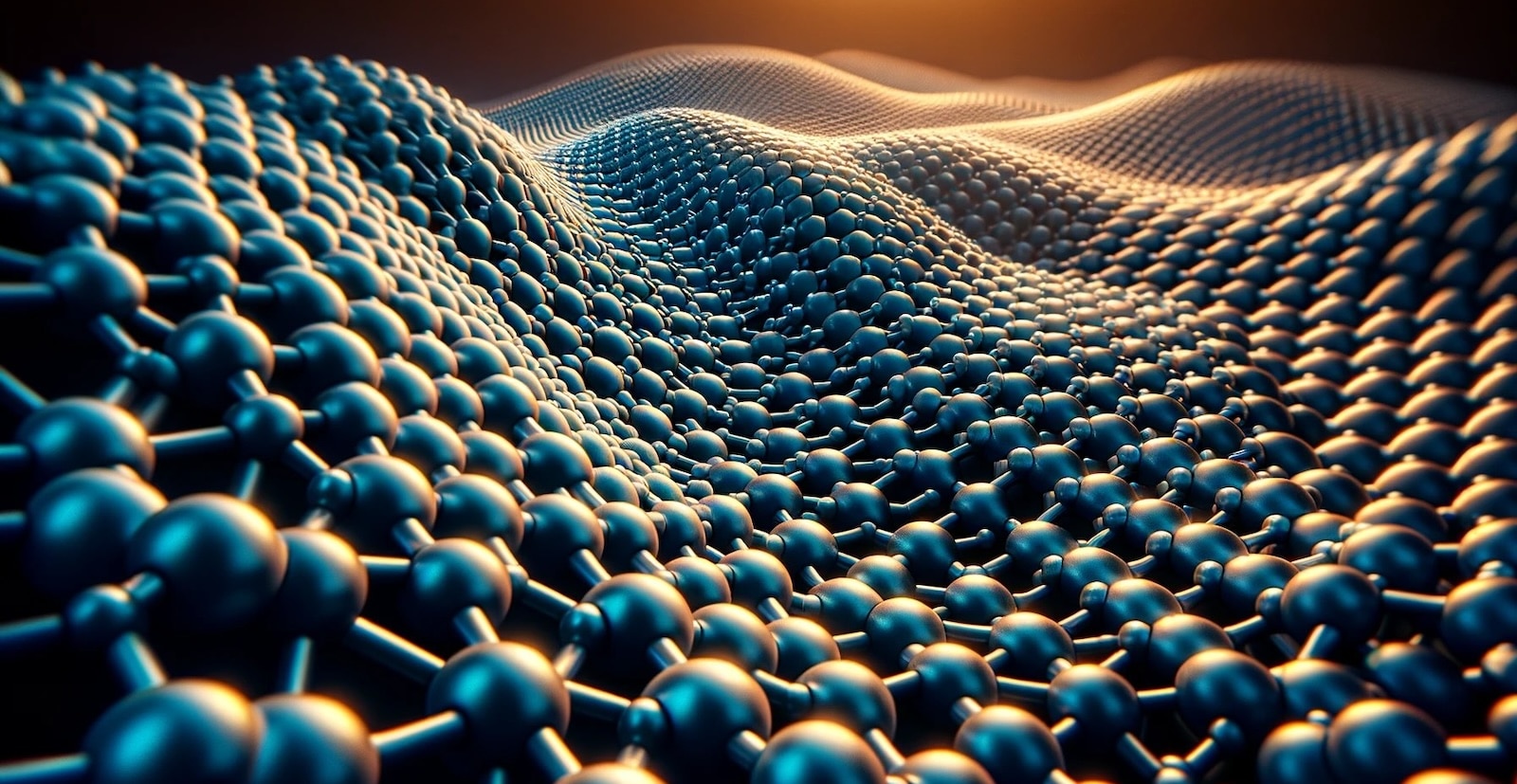
Powering Batteries with Nanotechnology
Nanotechnology involves working with very tiny materials, often at the scale of atoms and molecules, to create innovative and efficient products.
Scientists can enhance surface area, reactivity and conductivity by employing nanomaterials in battery components like electrodes and electrolytes.
This precise control at the nanoscale allows for more efficient energy storage and transfer, ultimately contributing to developing high energy density batteries that can power devices with increased performance and longevity.
Battery Basics: Past Progress and Future Potential
Batteries have evolved from the rudimentary, voltaic pile to advanced technologies, marking a trajectory of increased energy density, improved efficiency and diverse applications across industries.
Invented by Gaston Planté in 1859, the lead-acid battery was the first rechargeable battery and has been widely used in automobiles, uninterruptible power supplies (UPS) and renewable energy systems.
Nickel-cadmium (Ni-Cd) batteries were developed in the early 20th century and were commonly used in portable electronics and early cordless tools.
The alkaline battery was invented by Lewis Urry in the 1950s, providing a more reliable and longer-lasting alternative to zinc-carbon batteries. They are commonly used in consumer electronics.
The lithium-ion battery, developed by John B. Goodenough, Stanley Whittingham and Akira Yoshino in the 1970s, revolutionized portable electronics and later won a Nobel Prize. They are widely used in smartphones, laptops, and electric vehicles.
Introduced in the late 20th century, nickel-metal hydride (NiMH) batteries offered higher energy density than nickel-cadmium (Ni-Cd) batteries and became popular in applications like digital cameras and hybrid vehicles.
Anticipating the future, high energy density batteries, like solid-state and advanced lithium-ion, aim for increased capacity and sustainability.
High energy density in batteries is a transformative force for electronics and power storage, enabling smaller, lighter and more powerful devices with extended usage.
This revolution extends to renewable energy, enhancing the efficiency of storage systems and supporting the widespread adoption of electric vehicles by providing longer ranges and faster charging times, ultimately shaping a more sustainable and technologically advanced future.
Enter the World of Nanotech
Nanotechnology is changing how lithium-ion batteries operate by controlling things at the atomic level. This precision allows the use of nanomaterials in different parts of the battery, like electrodes and electrolytes,which improves performance and stability.
Creating new battery structures, such as nanowire-based anodes or nanocomposite cathodes, can enhance energy density, speed up charging times and extend the battery’s lifespan.
Nanostructured Silicon Anodes
Silicon nanowire battery electrodes offer a solution to common issues in batteries.
They can handle significant strain without breaking, ensuring efficient electronic contact and conduction. Silicon is attractive as it can hold ten times as many lithium ions by weight as graphite, but it tends to swell with charging and discharging, ultimately leading to early failure.
The technology improved with the introduction of carbon shell nanoparticles surrounding the silicon nanoparticles, allowing the silicon to swell and shrink, protected from the electrolyte.
Amprius hypothesized that a silicon anode made from nanosized silicon wires would solve these problems — and it did. The anode retained much of its theoretical energy storage capacity even with repeated charging and discharging cycles.
Nanoengineered silicon helps overcome swelling and shrinking during the charge and discharge cycle and gives lithium-ion batteries much more energy density than a conventional graphite anode. It was a win for nanotechnology and battery technology alike.
In December 2023, Amprius cut the ribbon on its silicon nanowire anode megawatt-hour manufacturing line, showing this technology’s promise. The factory will manufacture industry-leading, high-energy batteries, expected to deliver energy densities of over 500 Wh/kg and 1300 Wh/L and bring the company from kWh to MWh production scale.
Beyond Lithium-ion
Beyond lithium-ion, several emerging battery chemistries and technologies show promise for nanotech scaling.
Lithium-sulfur (Li-S) Batteries with Nanomaterials
Every sulfur atom can hold a pair of lithium, which means a lithium-sulfur battery could hold a lot of energy density.
Sulfur is an inexpensive material, but it poses some issues – it is not the ideal conductor and reacts with many common electrolytes, creating conditions that damage the longevity of batteries.
Sodium-sulfur is another battery technology under research, with University of Texas at Austin researchers recently taking this tech one step closer to reality. Sodium and sulfur are inexpensive and abundant, offering a potentially very affordable new battery solution with a longer life cycle.
Researchers in Sweden experimented with combining the cathode and electrolyte of a Li-S battery into one liquid, a “catholyte.” They then developed a nanoengineered graphene aerogel that acts as a free-standing electrode in the battery cell, allowing for better and higher sulfur utilization.
Nanotechnology in Cathodes
Lithium-sulfur-dioxide (LiSO2) batteries are known for their high energy density.
They are commonly used in applications such as military devices, communication equipment and some medical devices due to their long storage life and reliability.
Sodium-Ion (Na-ion) Batteries
Similar to lithium-ion batteries but with sodium ions, Na-ion batteries are a potential alternative for large-scale energy storage. They offer a compelling choice as they can be made on lithium-ion battery manufacturing lines, which means a reduced cost in infrastructure if these batteries become popular on a wide scale.
However, sodium ions are about 70 percent larger in radius than lithium ions, making their practicality challenging.
Researchers have developed crystalline nanowires which show promise for the construction of a viable sodium-ion battery system, particularly for grid-scale energy storage.
Solid-State Batteries
Solid-state batteries replace liquid electrolytes with solid materials, offering potential safety, energy density and lifespan advantages.
Nanotechnology is instrumental in designing nanoscale electrolytes and interfaces, improving ion conductivity and stability in solid-state batteries and addressing safety concerns associated with traditional liquid electrolytes.
Scientists are exploring the use of aluminum oxide (Al2O3), silicon dioxide (SiO2), and zirconium dioxide (ZrO2) nanoparticles added to solid polymer gel to enhance the conductivity and storage capacity of electrolytes.
Potassium-Ion Batteries
Like sodium-ion batteries, the technology for potassium-ion batteries is similar to lithium-ion batteries. However, potassium’s ionic radius also creates problems for energy storage and battery performance.
Researchers are considering NiCo2Se4, a bimetallic selenide, for creating spherical electrodes, creating a nanotube structure with channels for potassium ion and electron transfer.
Lithium-Air Batteries
Lithium-air batteries are of great interest as oxygen is a battery storage material available from the air around us. These batteries do not require metal oxide cathodes but instead generate their power from reactions with atmospheric oxygen.
However, like many other battery technologies still under investigation, there are drawbacks. For lithium-air batteries, the biggest challenge is energy waste and fast degradation.
Researchers at the Illinois Institute of Technology and the U.S. Department of Energy’s Argonne National Laboratory are innovating solid-state and lithium-air batteries with the potential to increase energy density by up to four times. The solid electrolyte is made from a ceramic polymer material with inexpensive elements in nanoparticle form.
Aluminum-Ion Batteries (Al-ion)
Aluminum was rejected as a battery material that could be useful with lithium-ion technology since it fractures and fails as it expands and contracts when exposed to lithium during charging and discharging. However, aluminum may be poised to play a critical role in solid-state electrolytes and nanotechnology.
Researchers in Australia created graphene aluminum-ion cells with nanotechnology from the University of Queensland’s (UQ) Australian Institute for Bioengineering and Nanotechnology. Nanotechnology is used to insert aluminum atoms inside graphene planes, with swift charging times, high power density and no cooling or heating issues.
Zinc-Air Batteries
Like lithium-air batteries, zinc-air batteries utilize the reaction between zinc and oxygen for energy storage. Zinc has commonly been used in one-time batteries but not rechargeable technology.
Still, a Canadian researcher used nanotechnology to make that possible, creating a nitrogen-doped carbon nanotube to use as a catalyst.
The resulting zinc battery has three to five times the energy density of lithium-ion batteries and it can be manufactured for one-sixteenth of the price, given the expense of lithium.
Researchers at Stanford University also did work in this field, using nanocrystals and carbon nanotubes to create a catalyst to improve the performance of zinc-air batteries. They used the nanomaterial on the cathode and achieved an energy density of >700 Wh/kg with a prototype battery.
The Power is in the Particle
The integration of lithium-metal anodes and novel cathode materials represents a groundbreaking frontier in battery technology and offers the promise of ultra-high capacities.
With their high theoretical capacity, lithium-metal anodes are essential to significantly enhancing energy density. Yet, they create serious safety concerns and offer a poor lifespan which hinders commercial applications of next-generation energy storage systems.
This includes many of those listed above, like Li-Air and Li-Sulfur batteries and emerging improvements in Li-ion batteries.
Traditional lithium metal anodes can develop dendrites during the charging process. Tiny, needle-like structures that grow from the surface of the lithium anode pose a risk of short circuits and reduced battery life.
Nanostructuring the lithium metal helps distribute stress more evenly, reducing the formation of dendrites. This arrangement enhances the safety and longevity of the battery.
Large lithium metal anodes can have limited surface area for lithium-ion interaction which affects the efficiency of charge and discharge processes. However, nanostructuring increases the surface area of the lithium metal, allowing for more lithium-ion interaction and improved energy storage capacity, faster charging and discharging and overall better performance.
Paired with innovative cathode materials, this combination aims to overcome challenges related to stability and efficiency.
By focusing on these advanced nanotechnology components, researchers are poised to usher in a future where batteries deliver power and endurance for a diverse range of applications across many industries.
Architecting for Energy
Layering active materials, controlling interfaces and maximizing surface area and density at the nanoscale level are pivotal strategies in architecting advanced energy storage systems.
This nanoscale approach allows for the precise arrangement of active materials, enhancing their reactivity and overall performance.
Precisely controlled interfaces contribute to the stability and efficiency of energy transfer within the battery, while maximizing surface area and density improves the overall storage capacity.
Researchers are engineering cutting-edge energy storage systems by adopting these nanoscale architectural principles.
Charging Into the Future
Despite ongoing challenges, the potential for high-energy, nanostructure batteries to revolutionize electric vehicles (EVs), grid storage and beyond is evident.
These batteries hold the key to unprecedented advancements in the efficiency, range, and sustainability of EVs, as well as the reliability and scalability of grid storage systems.
The path is clear: with further innovations, nanostructured batteries are on the verge of propelling us toward a cleaner and more energy-efficient future.
On the Horizon
The future of battery technologies is expanding with the integration of nanotechnology, offering novel solutions like quantum dots, hydrogen storage and innovative designs beyond lithium, as noted above.
Quantum Dots
Quantum dots, or nanoscale semiconductor particles, exhibit unique properties due to their small size and quantum mechanical characteristics.
Researchers are considering them, in particular, for use with rechargeable lithium-sulfur batteries, as they can modify the positive electrode and separator materials.
They provide a large surface area, offering active sites for absorbing and containing unwanted substances. This helps improve Li-S batteries’ performance by reducing unwanted effects during charging and discharging.
Researchers have experimented with different types of quantum dots to capture unwanted substances near the cathode, including TiO2 quantum dots grown on ultra-thin MXene nanosheets. These nanohybrids showed high conductivity, inhibiting any unwanted effects during battery operation.
Similarly, carbon dots and black phosphorus quantum dots have been explored for their ability to enhance the performance of Li-S batteries by improving the adsorption of unwanted substances.
Hydrogen Storage
Hydrogen storage involves the application of advanced nanomaterials to enhance the efficiency of storing and releasing hydrogen gas.
These nanoengineered materials, including nanocomposites, exhibit enhanced hydrogen sorption properties, addressing challenges such as slow kinetics and limited storage capacities.
The precision afforded by nanotechnology allows for the development of materials with optimized hydrogen release, contributing to the creation of compact, lightweight and efficient hydrogen storage solutions for clean energy applications, particularly in fuel cells.
Conclusion
As we observe and engage in the future of battery technologies, the integration of nanotechnology stands as a pivotal advancement that holds the promise of revolutionizing energy storage systems across industries. We may see unprecedented energy densities, efficiencies and lifespans, paving the way for a more sustainable and technologically advanced future. This highlights the potential for significant breakthroughs in electric vehicles and grid storage and also underscores the transformative impact of nanotechnology in helping craft the next generation of energy solutions.
